Remote sensing of water
Tags :: Remote Sensing
Water Quality
Monitoring water quality is crucial to ascertain the sustainability and usability of water. The physical, chemical, and biological characteristics of water determine its quality and are traditionally measured through field and laboratory analysis. Remote Sensing is one method of collecting data at scale.
Spatio-temporal characteristics are influenced by several factors such as:
- Location of sampling
- Urbanization
- Land-use transitions
- Deforestation
- Climate change (Temperature change in particular)
As surveys over large areas spanning long periods become infeasible, satellite and airborne sensors provide valuable information.
The spectral characteristics of a clear water surface are significantly different from those of water mixed with impurities/pollutants. Clear water absorbs 97-99% of incident energy and reflects 1-3% of incident radiation.
Polluted water has higher reflectance.
The dominant reflected wavelength changes with the change in constituents in the water, resulting in unique spectral signatures.
To monitor water quality, a spectral relationship between spectral reflectance and water quality parameters is established with the general relationship as:
\[ Y = A + BX \quad \text{(or)} \quad Y = AB^X \]
where \(Y\) is the spectral reflectance; \(X\) is the water quality parameter of interest, and \(A\) and \(B\) are empirical factors.
Water with low sediment concentration has a maximum reflection of \(0.55 \mu m\) compared to \(0.6 \mu m\) by water with high sediment concentration (Ritchie et al.).
Chlorophyll-a
Chlorophyll-a is one of the most widely examined parameters using remote sensing information. It is a photosynthetic pigment responsible for causing the green color in plants.
Its presence in water is directly linked to the occurrence of water algal blooms.
Nitrogen and phosphorus, often from fertilizer runoff and the burning of fossil fuels, catalyze growth.
The spectral reflectance curve for chlorophyll-a in water shows:
- Absorption:
- Blue \(\sim 0.4 \mu m\)
- Red \(\sim 0.7 \mu m\)
- Reflectance:
- Green \(\sim 0.5 \mu m\)
- NIR \(\sim 0.8 \mu m\)
Studies have found it challenging to determine Chl-a concentration in water bodies with high suspended sediments.
Landsat TM and ETM+ are widely used for Chl-a monitoring.
Formula to determine Chl-a content empirically using band ratio (Harding et al.):
\[ \log_{10}[Chl - a] = A + B( - \log_{10} G) \]
where:
\[ G = \frac{(R_2)^2}{R_1 \times R_3} \]
\(A\) and \(B\) are constants from in situ measurements, and \(R_{1,2,3}\) are the spectral reflectance at 460 nm, 490 nm, and 520 nm, respectively.
Other empirical algorithms include 2BDA, 3BDA, normalized difference chlorophyll index (NDCI), maximum chlorophyll index (MCI), etc.
Colored Dissolved Organic Matter (CDOM)
Also referred to as aquatic humus, limnohumic acid, gelbstoff, or gilvin.
CDOM consists of macromolecules with aromatic, carboxylic, fulvic, and humic acids derived from the decomposition of plant material in soils and wetlands, bacterial activity, algal growth, and sediment loading.
When present in high concentrations, CDOM imparts a yellow-brown color to the water.
Higher CDOM levels attenuate light penetration into water bodies and support the growth of phytoplankton.
CDOM is found to be strongly correlated with Chl-a, TSS, and turbidity levels.
Visible spectral bands are relevant in monitoring CDOM concentration.
CDOM presence causes absorption in the ultraviolet range and blue-green bands.
Several models exist for determining CDOM levels.
Surface and Subsurface
Streamflow Estimation
River discharge is the volume of water flowing through a river cross-section per unit of time.
Satellite remote sensing can circumvent the challenges of in situ analysis while estimating discharge and storage.
Since streamflow discharge cannot be measured directly by satellite sensors, other hydraulic variables such as water level height, velocity, river cross-sectional area, river width, and surface water slope are measured by sensors.
Radar altimeters are useful for estimating water level variations in inaccessible areas. Altimeters measure the time taken by radar pulses to cover a round trip from the satellite to the water surface, which is then used to determine the distance from the satellite to the water surface. The difference in water level is computed by calculating the differences in the satellite’s position with respect to the reference ellipsoid and the distance from the satellite to the water surface.
The general equation to measure the height of the water surface from the satellite is:
\[ H = h - R + corr \]
where \(H\) is the water surface height; \(h\) is the height of the satellite orbit; \(R\) is the altimeter range measurement, and \(corr\) represents the various corrections pertaining to Tropospheric (\(TC\)), Ionospheric (\(IC\)), and Tidal (\(T\)) corrections.
\[ corr = TC + IC + T \]
Index Table
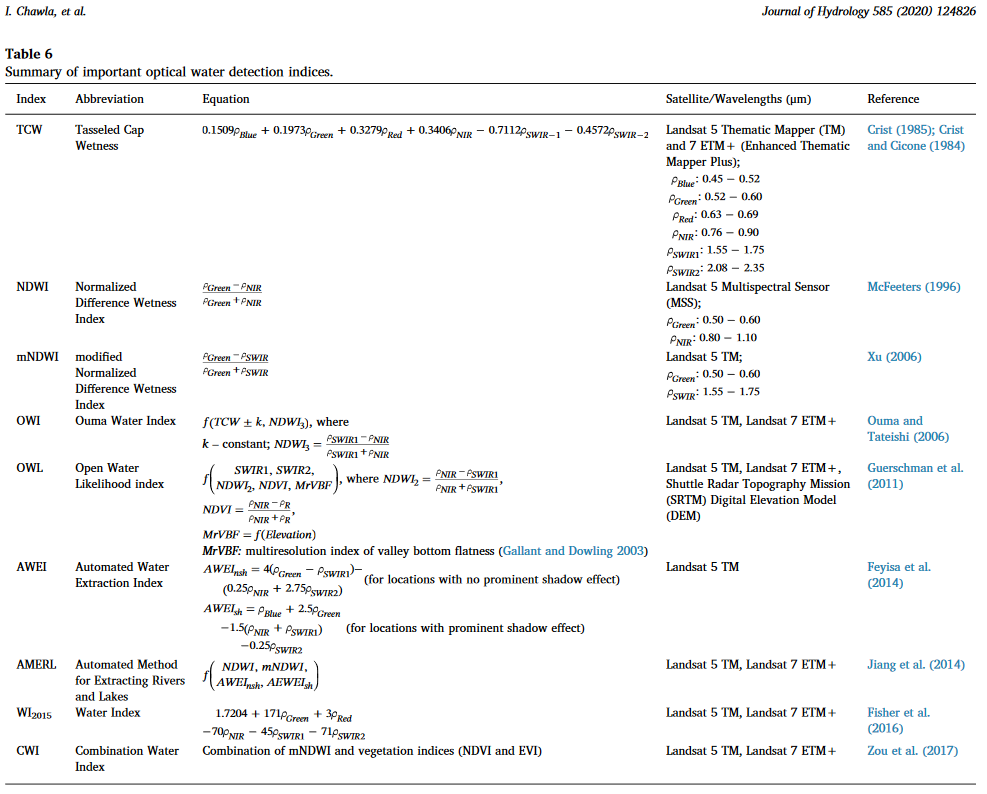